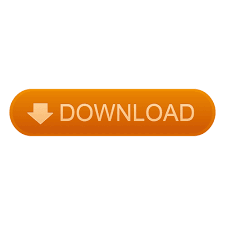
The volume fraction of the probe particles was varied between 6 × 10 −11 and 6 × 10 −5 yielding identical transport properties of the studies solutions. Hence, the ensuing error in the measured solution transport properties is small. The corresponding increase of the particle radius is up to 7 nm, i.e., much less than the original particle size. Adhesion of the positive lysozyme molecules-lysozyme's pI is 11.5-on the particles would likely be limited to one or two layers. This prevents their aggregation due to depletion attraction induced by lysozyme. 14), so that at our experimental pH = 7.8 the probe particles are negatively charged. The probe particles are coated with bovine serum albumin (BSA). This 〈 r 2〉( τ) was determined by dynamic light scattering from the probe particles of radius R 0 = 300 nm (see below for determination of R 0). To determine if the hydrodynamic response in the tested solutions is purely viscous or partially elastic, we record the correlation between the mean squared displacement 〈 r 2〉 of probe particles and the observation time τ. The results are output as concentration of clusters of certain size as a function of this size. The clusters sizes are determined using the Einstein-Stokes relation and the solution viscosity determined as discussed below. The diffusion coefficients of the individual clusters are computed from the trajectories indicative of regular Brownian motion of the imaged cluster.
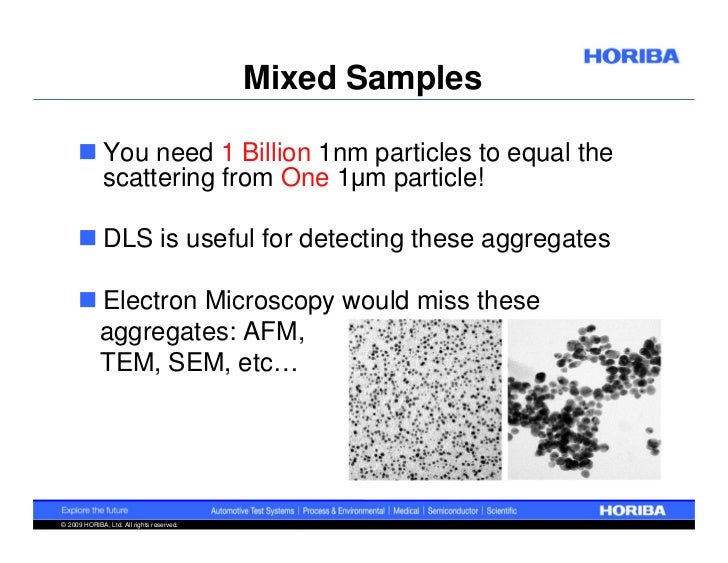
The Brownian trajectories of the clusters are tracked by comparing the locations of the individual clusters in a sequence of images collected at a rate 30 s −1. Each cluster is treated as a point source of scattered light and its location is determined from images such as the one in Fig. Since the protein molecules are significantly smaller than the clusters, the light scattered from them is insignificant even at relatively high protein concentrations. This device detects the light scattered off the clusters by a camera attached to a microscope. Data are collected from a solution volume 120 × 80 × 5 μm 3 (width × length × height). The protein solution, held in a custom-made cuvette, is illuminated by a specially configured laser beam. We use a Nanosight LM10-HS device optimized for determination of protein aggregates. DLS yields a lower bound of the cluster lifetime, which may be orders of magnitude lower than the real one.
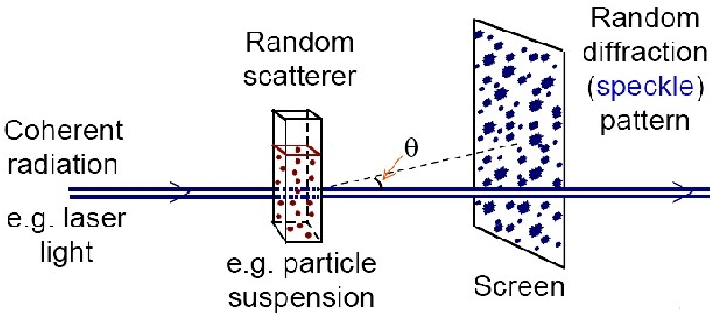
We show that DLS yields a reliable width of the cluster size distribution only if the cluster concentration is above 10 9 cm −3 and their volume fraction is above 10 −6.
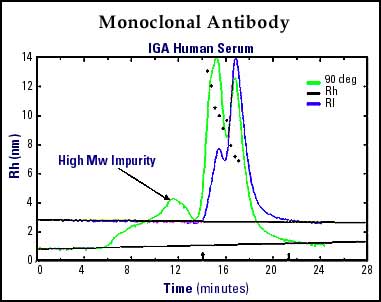
We put forth an alternative method to determine the aggregates’ sizes, concentrations, and volume fractions. The CONTIN algorithm, often employed to process DLS data, may, in some instances, produce non-physical results. The related underestimate of the cluster concentration is ∼10 ×. The factor of overestimation depends on the shape of the size distribution and is ∼1.6 × in the studied solution. We show that because of the sixth power dependence of the scattered light intensity on the size of the scatterers, DLS overestimates the mean size of the clusters. We compare the results of DLS to those of Brownian microscopy. We use as an example a solution of the protein lysozyme in which dense liquid clusters of radius about 100 nm reproducibly exist.
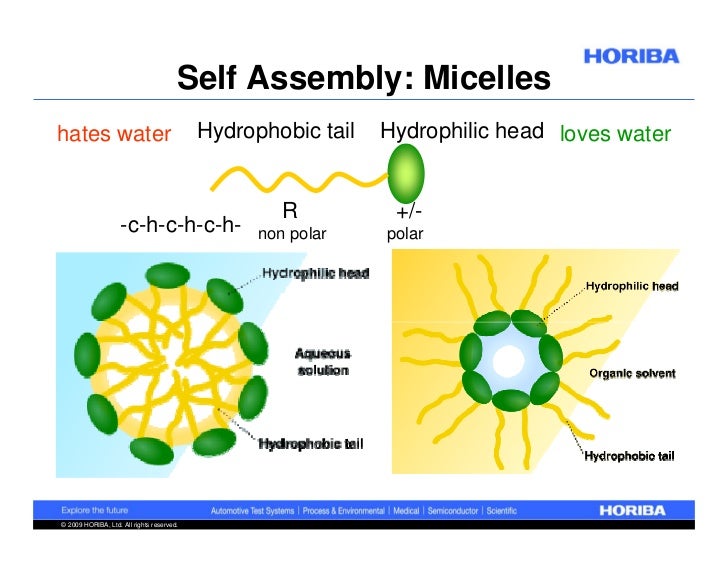
Here, we explore the veracity of the aggregate sizes, size distribution widths, concentrations, and lifetime resulting from DLS. Dynamic light scattering (DLS) is often used to monitor aggregation in protein solutions.
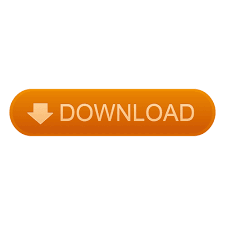